The role of RAN translation in neurodegeneration
About Project
Prot-RAN: What drives the RAN translation and why do we get neurodegenerative disease when it goes wrong?
The short tandem repeats, usually three to eight base pairs long, are common in human genome. They are also genetically unstable and their uncontrolled expansion may lead to inherited disorders. For example, in the 5’UTR of fragile X mental retardation 1 (FMR1) gene, healthy individuals possess typically between 5 and 54 CGG trinucleotide repeats, while premutation expansions (55-200 CGG repeats) causes fragile X-associated tremor/ataxia syndrome (FXTAS), and a full mutation (above 200 repeats) leads to fragile X syndrome (FXS). To date, more than 40 human inherited disorders were linked to the expansions of simple repeat sequences, mostly trinucleotide repeats. Among those typically multisystem diseases are Huntington’s disease (HD), myotonic dystrophy and mentioned above FXS and FXTAS.
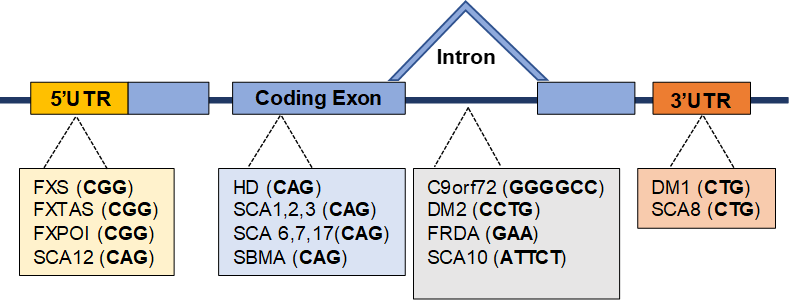
Figure 1. Examples of the distribution of triplet repeats responsible for particular diseases within the genome. Disorders are listed by their genomic location on a schematic gene. Abbreviations: Fragile X Syndrome (FXS), Fragile X-associated tremor/ataxia syndrome (FXTAS), Fragile X-primary ovarian inusifficiency (FXPOI), Spinocerebellar ataxia (SCA), Huntington’s Disease (HD), Spinal-bulbar muscular atrophy (SBMA), Myotonic dystrophy (DM), Friedreich ataxia (FRDA).
In Prot-RAN project, we will focus on the expansion of trinucleotide CGG repeats (CGGexp) in the 5’UTR of FMR1 gene, which causes common neurodegenerative disease, FXTAS. The pathogenesis of FXTAS remains unclear, and to date, various pathogenesis models have been proposed. One of the possible mechanism is the repeat associated non-AUG initiated (RAN) translation. This phenomenon is based on the observation that the expanded short tandem repeats can trigger the production of mutant proteins, without the canonical AUG initiation codon, which is usually used for protein translation. Resulting aberrant proteins accumulate in nuclear inclusions in the brain of FXTAS patients, leading to neuronal death.
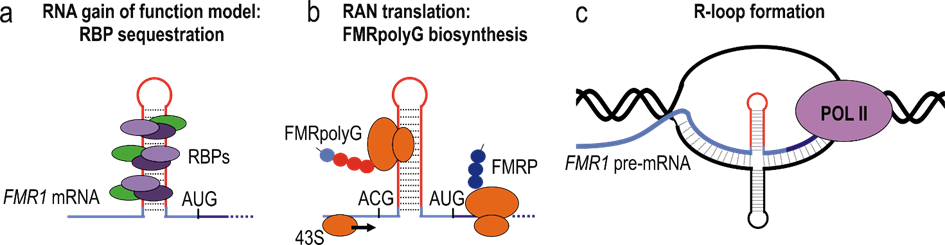
Figure 2. Possible pathogenesis models of FXTAS. CGG repeats (red line) within 5`UTR of FMR1 mRNA (blue line) create stable RNA secondary structure which induces the pathologic effects: a) sequestration of specific RBP proteins in nuclei, b) the non-canonical FMRpolyG protein biosynthesis and c) excessive R-loop formation during transcription (interaction between DNA (black) and complementary RNA strands).
RAN translation
The translation of short tandem repeats non residing in the canonical AUG-initiated open reading frame, into proteins was initially described in 2011 in SCA8 and in DM1. So far, RAN translation has been confirmed in nine different pathologies: SCA8, DM1, DM2, HD, FXTAS, C9orf72 amyotrophic lateral sclerosis and frontotemporal dementia (C9 ALS/FTD), fragile X premature ovarian insufficiency (FXPOI), spinocerebellar ataxia type 31 (SCA31), and Fuchs endothelial corneal dystrophy (FECD). The prevalence of RAN translation across different microsatellite motifs suggests that it may be a shared mechanism in most microsatellite expansion disorders.
Emerging studies reveal that the non-AUG translation initiation is more common than previously thought, as the translation can be initiated at codons that differ from canonical AUG codon by a single nucleotide, such as CUG, GUG or ACG. Although these event are relatively non-efficient, they play important role, i.e. translational control when encoding upstream open reading frames (uORFs), production of functional proteins (i.e. DAP5) and can promote disease progression, such as cancer or neurodegeneration.
In FXTAS, the CGGexp initiates the RAN translation mechanism in the 5’UTR of FMR1 mRNA, producing polyglycine (FMRpolyG) and polyalanine (FMRpolyA) proteins in the GGC and GCG reading frames, respectively. The FMRpolyG translation is the most efficient, and initiates at the near-cognate ACG and GUG codons located upstream of the CGGexp. The translation initiation of FMRpolyA probably occurs within the repeats (GCG codon), with ~30% efficiency of FMRpolyG translation. Moreover, the FMR1 locus can be also transcribed in opposite direction, producing an antisense transcript, ASFMR1, and can generate polyproline (ASFMRpolyP), polyalanine (ASFMRpolyA) and polyarginine (ASFMRpolyR) products.
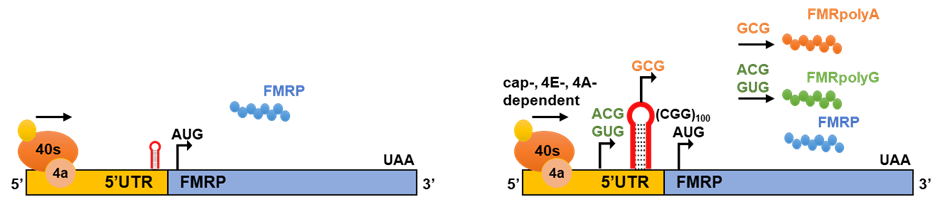
Figure 3. Production of RAN translation proteins at CGG repeats in FMR1 from multiple reading frames. a) Canonical translation in FMR1 mRNA occurs in unaffected individuals with <50 CGG repeats. b) Expanded CGG repeats trigger initiation of RAN translation in multiple reading frames, using 5’-cap, eiF4E- and eIF4A-dependent scanning mode. ACG, GUG reading frames leading to production of FMRpolyA and GCG reading frame leading to production of FMRpolyG are shown.
Objectives & Methods
Despite emerging reports about the possible mechanisms driving RAN translation, still little is known about this process. The main goal of the Prot-RAN project is to identify proteins regulating RAN translation, which will help to understand the disease mechanisms and find potential drug targets for neurodegenerative diseases- FXTAS, HD and other short tandem repeat expansion disorders.
To achieve this goal, we will bridge cutting-edge proteomics with RNA biology techniques. Briefly, we will employ the CGGexp RNA-targeting pull-down approaches combined with proteomic profiling, RNA mutagenesis, protein expression analysis and RNA/protein interaction studies. The role of identified proteins will be then verified in other expansion disorders, e.g. HD, giving insight into more global context of the RAN translation. As a result, the discovered factors driving RAN translation could be used as potential new targets for therapeutic strategies of microsatellite expansion disorders.
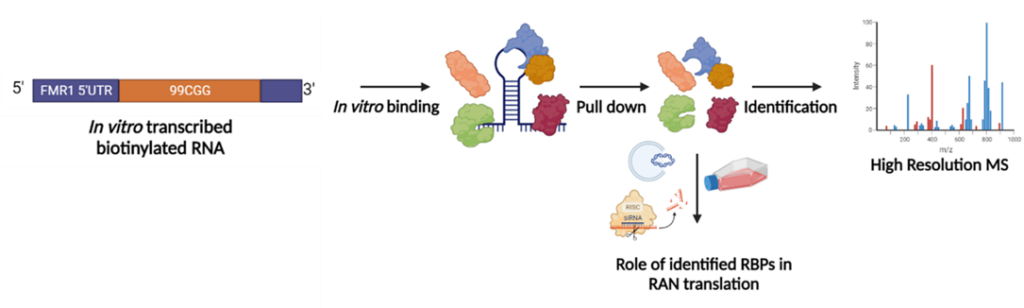
Figure 4. General work plan of the Prot-RAN project.
Research group information
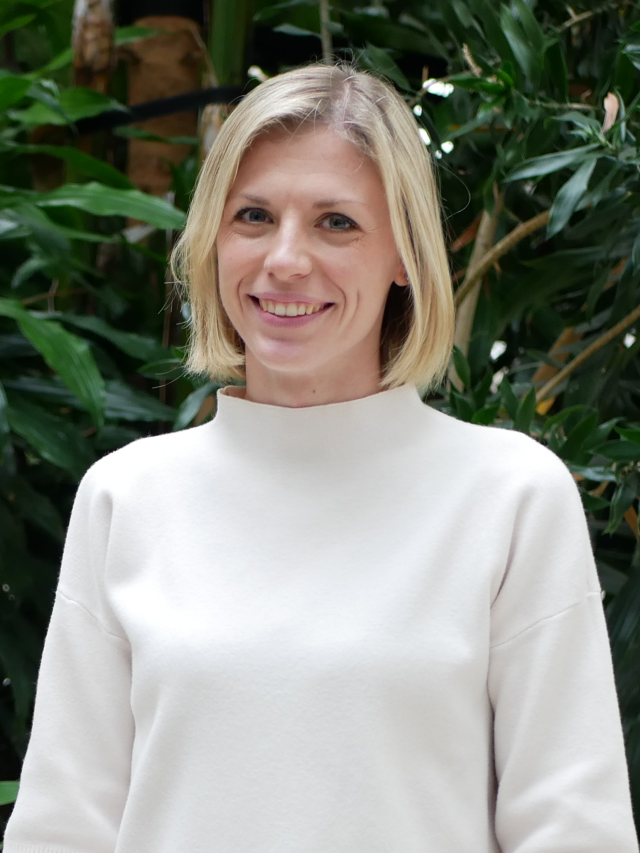
Anna Baud is an experimental biologist and her favourite techniques are proteomics and mass spectrometry. She received her MSc from Westpomeranian University of Biotechnology in Szczecin, and did her PhD in the field of structural and functional biology at University of Evry, France. She then completed two post-doctoral trainings in the field of translational mass spectrometry: at University College London (UK) and University of Lille (France) which allowed her to develop further her analytical skills and deepen her knowledge about proteomics. In January 2019, Anna was appointed to Post-Doc position in the TEAM project in Prof. Sobczak lab, and then received a prestigious Marie Skłodowska-Curie Widening Fellowship. In her research, she bridges her experience in proteomics with RNA biology and neurodegenerative diseases, and is generously supported by H2020 MSCA IF and NCN Sonata-15 grants.
Contact information:
Email: | anna.baud@amu.edu.pl |
Phone (AMU landline): | +48 61 829 5952 |
AMU Research portal: | https://researchportal.amu.edu.pl/info/author/UAM223184/ |
LinkedIn: | https://www.linkedin.com/in/anna-baud-52a9327a/ |
ResearchGate: | https://www.researchgate.net/profile/Anna-Baud |
Funding information:
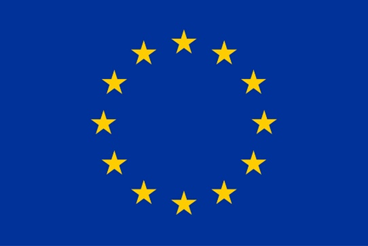
This project has received funding from the European Union’s Horizon 2020 Research and Innovation Programme under the Marie Sklodowska-Curie grant agreement No. 101003385 and Polish National Science Centre No. 2019/35/D/NZ2/02158.
Selected Publications:
- Baud A., Derbis M., Tutak K., Sobczak K. Partners in crime: Proteins implicated in RNA repeat expansion diseases. WIREs RNA. DOI: 10.1002/wrna.1709. (I.F. 9.957) https://wires.onlinelibrary.wiley.com/doi/full/10.1002/wrna.1709
Selected Conferences:
The 5th international conference on FMR1 premutation: molecular mechanism, clinical involvements and target treatments.
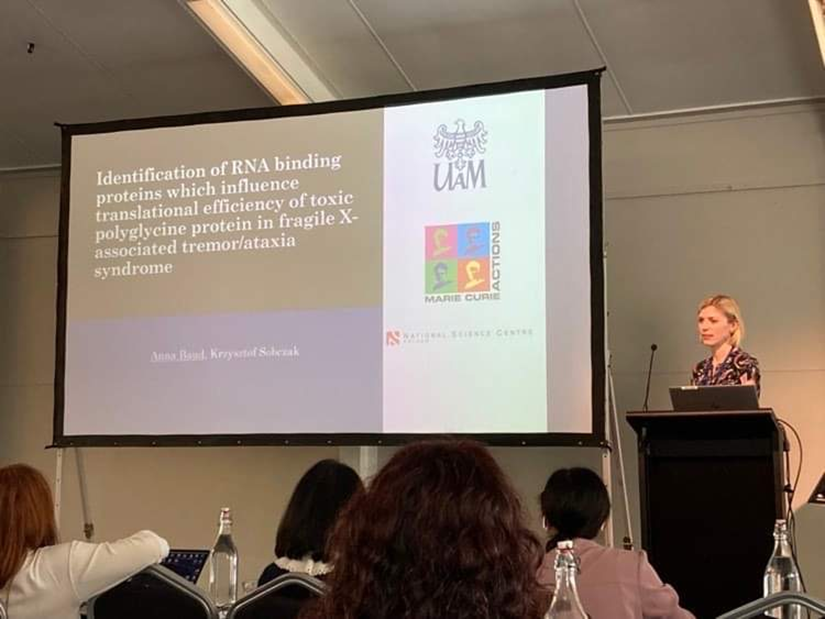